Genuine trackers/hodoscopes (large area muon systems, inner tracking/vertexing)
WP Leaders:
- Giulio Aielli
- Riccardo Farinelli
- Mauro Iodice
- Atsuhiko Ochi
- Gabriella Pugliese
Contact: DRD1-WP1-leaders@cern.ch
Participating institutes:
CEA/Saclay IRFU, CERN, CIEMAT, IFIN-HH, INFN Bari, INFN Bologna, INFN Ferrara, INFN Laboratori Nazionali di Frascati, Hong Kong University (HKU), Chinese University of Hong Kong (CUHK), Hong Kong University of Science and Technology (HKUST), INFN Napoli, INFN Roma Tor Vergata, INFN Roma Tre, INFN Torino, Institute of General and Physical Chemistry (IGPC), Istinye University (ISU), Kobe University, Ludwig Maximilian University of Munich, Max Plank Institute for Physics, National University of Science and Technology Polytechnic Bucharest (UNSTPB), University of Cambridge, University of Geneva, University of Oviedo and ICTEA, University of Transylvania Brasov (UniTBv), USTC, Vrije Universiteit Brussel (VUB), Weizmann Institute of Science, Wigner RCP, Brookhaven National Laboratory, Florida Institute of Technology, Jefferson Lab, Michigan State University, Tufts University, University of California Irvine, University of Massachusetts Amherst, University of Florida, University of Michigan, University of Wisconsin.
Institute contacts: DRD1-WP1-Institute-Contact@cern.ch
DESCRIPTION OF THE WORK PACKAGE
The primary objective of the project is to strategically advance R&D in the domain of resistive gaseous detectors for applications as trackers, hodoscopes, and large-area muon systems for new challenges at future facilities. The goal is to strengthen their stability, robustness, and long-term performance, as well as to optimize a cost-effective manufacturing together with industrial partners.
In view of applications at future electron colliders (ILC/C3, FCC-ee, CepC), Muon collider, Hadron Physics, FCC-hh, the main challenges for future muon systems include the following:
-
Extending the state-of-the-art rate capability by at least one order of magnitude up to ~1-10 MHz/cm2 with longevity compatible with decades of operation. This involves advancements in detector resistive configurations, new materials and geometries for improved signal pick-up, low-noise electronics, and fine granularity readout to reduce occupancy.
-
Enabling reliable and efficient operation with low-GWP (Global Warming Potential) gas mixtures.
-
Improving time resolution at the level of nanosecond and achieving resolutions up to 10-100 ps for applications in high-rate collider experiments to mitigate pile-up effects.
-
Establishing large-scale serial production and cost reduction measures.
-
Addressing low/medium-rate applications involving muon tracking in HEP experiments like at FCCee, and exploring applications beyond HEP for large areas (in connection with WP9)
Main drivers from the facilities
Gaseous detectors will remain the primary choice for muon tracking and triggering at future facilities whenever cost-effective, large-area coverage with low material budget and high detection efficiency is required. Moreover, muon systems are often designed to provide a precise momentum measurement, usually in combination with an inner tracker. Precision nanosecond-level timing also helps to mitigate pile-up effects and to reduce uncorrelated beam-induced backgrounds, while improving the sensitivity for heavy long-lived particle searches (e.g. slow muon-like particles with β < 0.9).
For HL-LHC operation from 2026 onwards, the CMS muon system will be upgraded by installing new RPCs and GEMs in the high eta region to restore the redundancy in the CMS Muon System and extend the eta coverage with the new ME0 station.
Several solutions (μ-RWELL), Micro Pixel Chamber (μ-PIC) and small-pad resistive Micromegas were initially considered for the very forward muon tagger in the ATLAS Phase II Upgrade Muon TDR proposal. Here, the main challenges are discharge protection and miniaturisation of readout elements, which can profit from the ongoing developments on Diamond-Like Carbon technology. The μ-RWELL approach is also considered as one of the tracker options in LHCb beyond LS4.
Gaseous detectors are also proposed for the future CBM and PANDA experiments at the FAIR facility. A GEM-based muon tracker with zigzag readout strips is foreseen at NA60+. Zigzag strips can cover a readout area with fewer strips than regular straight ones while maintaining good spatial resolution (∼ 100 μm) and rate capability (10 kHz/cm2). Addressing the short-term R&D needs for the existing muon systems beyond 2023 will require improvement of operation procedures to guarantee their longevity and stable, discharge-free, operation at high rates, and mitigation strategies for the use of greenhouse gases when it becomes mandatory (DRDT 1.3).
Muon systems at future e+e− colliders (ILC, FCC-ee, CLIC, SCTF) or LHeC do not pose significant challenges in terms of particle fluxes and radiation environment. The IDEA detector layout concept at FCC-ee will require operation at rates up to 10 kHz/cm2, spatial resolution of ~60−80 μm and a time resolution of 5-7 ns, with the total integrated charge < 100 mC/cm2. Currently available technologies (RPC, sMDT, MPGD) can be used to instrument large-area O(1000 m2) FCC-ee muon detectors.
The ILC muon system/tail catcher technologies can include RPC or MPGD, as for Hadron Calorimetry (HCAL). Some engineering challenges are related to the operation in the high magnetic flux return (up to several Tesla). Generally, background rates in LHeC muon detector, which is based on the updated design of ATLAS Phase II Muon spectrometer, are lower than in pp colliders.
The muon tracking and triggering at a future hadron collider, such as FCC-hh, also requires large area coverage (~3000 m2), while particle rates do not exceed 0.5 kHz/cm2 in the barrel and below 500 kHz/cm2 in most of the endcaps. The existing technologies for HL-LHC (MPGD, RPC, sMDT, sTGC) are adequate in most of these muon spectrometer areas. However, major R&D would still be needed for the very forward endcap region at a radius below 1 m.
In a multi-TeV muon collider, the Beam Induced Background (BIB), due to muon decays, dominates in the endcap region, reaching a maximum flux of O(MHz/cm2).
The main challenges at future facilities, particularly beyond 2030, include large area coverage with precision timing information (DRDT 1.1) to ensure correct track-event association, and the ability to cope with large particle fluxes using environmentally friendly gas mixtures (DRDT 1.3).
Key technologies
To meet these challenges, the project will leverage various technologies, including the RPC family with different variants (surface resistivity, multi-gap, thin-gap), the rapidly evolving and promising MPGD family (such as Micromegas, GEM, micro-RWELL, gridPix, micro-PIC), as well as hybrid structures combining innovative resistive structures with robust, reliable, wire structures (DT). For MPGDs, the main challenges remain large area, high rate, precise timing capabilities (PICOSEC, FTM), and stable discharge-free operation. They are addressed by developing resistive electrodes and exploring novel materials, amplifying structures, and detector architectures. Precise assembly at an industrial scale and integration of large area detector systems are additional items to be faced.
Relevance in context of ECFA Roadmap
As part of our long-term vision, we aspire to align the projects of WP1 with the requirements outlined in the 2021 ECFA detector research and development roadmap. Specifically, we aim to address the facility needs and recommendations set forth by ECFA, focusing on the following Detector Research and Development Themes:
DRDT 1.1 - Improve time and spatial resolution for gaseous detectors with long-term stability.
DRDT 1.3 - Develop environmentally friendly gaseous detectors for very large areas with high-rate capability.
TASKS AND DELIVERABLES
Addressing the need for large surfaces, the project will focus on cost-effective detector manufacturing, modularity, radiation-hard front-end developments for affordable and high-density channel readout, and fast, scalable multichannel data acquisition systems. The DRD1-WP1 includes eight tasks dedicated to tackling the future challenges.
T1: New RPC structures
This task aims to develop and optimize the RPC using new structures and materials to enhance its rate capability and timing resolution.
RPCs are highly valuable gaseous detectors known for their rapid timing and substantial detection area (up to square meters), all achieved at low costs. As a result, they find extensive application in numerous collider experiments, primarily for the precise timing and broad coverage of muon detection. However, the evolving demands of future high luminosity collider experiments are prompting the need for enhanced rate capabilities and faster timing resolution.
The core technologies crucial for enhancing RPCs to meet these requirements encompass the use of resistive materials and novel structural design. Among these, Diamond-Like-Carbon (DLC) deposition stands out as a promising technique. Additionally, ongoing research explores low-resistivity glasses and semiconductors as potential avenues for advancing RPC technology. Incorporating fine structures, such as narrow gaps and optimized electrode patterns, holds significant promise in achieving both heightened counting rates and refined timing resolution.
The goals of this task are to achieve RPCs with a high-rate capability (ranging from 10 kHz to 1 MHz per cm2) and/or improved timing resolution (reaching sub-ns to ps levels). Moreover, new RPC structures employing multi-gap and multi-channel readout configurations will be devised for space-time tracking purposes. The processes of prototyping and construction hold significant importance in this endeavour.
Deliverables:
-
D1.1: Design, construction, and tests of single/multi-gap surface resistivity RPC with DLC electrodes.
-
D1.2: Design, construction, and test of innovative RPC structures with current (HPL, glass) and novel resistive materials (phenolic glass, GaAs, etc.) working comfortably up to 100 kHz/cm2.
-
D1.3: Design, construction, and test of prototypes of RPC tracker structures (made of multiple independent gas gaps and readout electrodes) suitable for 3D space-time tracking, with millimetric space resolution and up to 100 ps time resolution.
T2: New Resistive MPGD Structures
This task focuses on developing and optimizing new resistive MPGD concepts and architectures, alongside consolidating existing solutions for large surface muon detectors. Key goals include achieving stable operation at high-gain for extended periods, large size, high-rate capability, precision time and spatial resolution, and cost-effective production.
Additionally, the task involves the development of accurate modelling tools, capable of driving the detector design in terms of induced signal and detector performances (space and time resolutions, rate capabilities, stability).
Optimization efforts extend to the readout configuration, aiming to reduce detector occupancy and handle the increased number of readout channels.
The task will conduct R&D on Diamond-Like Carbon (DLC) production processes to enhance uniformity and stability.
Furthermore, the task aims to optimize the size and modularity of the detectors to enhance their efficiency, and externalize the production to industries to promote scalability and cost efficiency.
Lastly, it will be essential to perform comparative studies to evaluate the advantages and critical points of different MPGD technologies.
Deliverables:
-
D2.1: Large area (approximately 50x50 cm2) prototypes based on resistive elements and novel MPGD architectures for high particle rates (range 100 kHz/cm2 – few MHz/cm2), 2D readout, < 100 μm space resolution, about 10 ns timing resolution, high gain (above 104) to ensure stability and providing a good margin for nominal working point.
-
D2.2: Large area MPGD prototypes for low/medium rates (from few to 100 kHz/cm2) for large surface coverage O(1000 m2), optimized in terms of performance (precise tracking – below 100 μm and timing – few ns) and overall cost.
-
D2.3: Support and update of the modelling and simulation framework for the study of induced signals in detectors with resistive elements.
T3: New Front-end electronics
Performance of detector technologies at the base of large muon trackers includes micrometric space resolution and time resolution up to tens of ps. Given their typically large dimension the capacitance associated with a single readout electrode cannot be easily reduced and it is non-trivial to impose that different points on the grounding shielding are equipotential in the frequency range of the fast pulses generated by the detectors. This situation imposes severe and competing constraints on the design of the front-end electronics integrated within the detector readout electrodes and grounding, in terms of S/N. On the other side, designing the next generation of muon detectors with a much higher counting rate capability and compatibility with ECO-gases, would greatly benefit from transferring part of the amplification from the detector to the FE, by exploiting an overall S/N as high as possible. S/N is also crucial to exploit the ultimate precision performance mentioned above. A second purpose in this development is to integrate a high-resolution digitization in the FE (principally TDCs and ADCs), so avoiding the transmission of analog information to a remote the readout system, would help to preserve pulse information content. Finally, in order to cope with the requirements of primary trigger systems, the information must be provided with a low and possibly fixed latency.
Deliverables:
-
D3.1: Design, production, and validation of a new ASIC chip for MPGDs based on a new architecture for the MPGD and a technical note about the chip expected performance.
-
D3.2: Integration and performance assessment of a Bi-CMOS SiGe heterojunction technology FE ASIC, with various detectors technologies, and Design of an increased performance ASIC with ultimate time resolution (< 10 ps) and possibility of local zero suppression and daisy chaining
-
D3.3: Development of a radiation hard multichannel TDC board and integration in a detector setup
T4: Optimization of scalable multichannel readout systems
Large muon tracker readout systems can be divided in two classes of applications: high-rate experiments at colliders and low-rate experiments for rare events searches. The former need a high granularity and low latency readout the latter can greatly reduce the granularity of the readout by exploiting the expected sparsity of the signal, by daisy-chaining FE channels using buffering and zero suppression techniques. Present scalable readout systems are based on modern FPGAs used as multi-channel serial receivers, or digitizing samplers, and for collecting and transmitting data to the acquisition system. While the use of FPGA systems seems to be a convenient choice at least in the short-mid term, it may be possible that the development of dedicated circuits working, as dedicated serial line receivers and signal pre-treatment, will be needed to overcome the limitations inherent to a general-purpose architecture such as the FPGA architecture.
Deliverables:
-
D4.1: Design, implementation, and optimization of novel Scalable Readout Systems for Gaseous Detectors
-
D4.2: Development of scalable high-speed processing and interface FPGA IP blocks for the DAQ ecosystem.
-
D4.3: Development of scalable DAQ software for high-speed data transport
T5: Eco-friendly gases
Some gaseous muon detectors use fluorinated gases(F-gases), such as the C2H2F4(TFE), SF6 and CF4, to ensure good detector performance, operation stability and longevity. However, these F-gases are considered non-eco-friendly due to their high Global Warming Potential (GWP), which represents the measurement of the greenhouse impact of a gas normalized to the CO2 (GWP =1). The search for a new ecological gas mixture is mandatory in order to both reduce the GHG emission from the detectors at the future accelerator and avoid risks of gas cost escalation and supply problems.
Long term performance studies together with fluoride production of the eco-friendly mixtures under analysis and the evaluation of their flammability will be performed in continuity with the work carried on in the framework of AIDAInnova project, and in full synergy with DRD1 WP1-T7
Deliverables:
-
D5.1: Test and characterization of RPCs operated with low-GWP (HFO) and new eco-gas mixtures.
-
D5.2: Compatibility of the new eco-gases with gas system components
T6: Manufacturing
The construction of large-area detectors at low cost is a general key point for all MPGD-based detectors. Technology transfer relations with industries allow to optimize the manufacturing procedure and to minimize time consuming or costly steps. The production of resistive micromegas and micro-RWELL is mandatory for the future detector production. We aim to study a modular design and an improvement of the detector technological aspects to produce large area (up to about 100 x 50 cm2) detectors to keep the production costs as low as possible, to share the detector production knowledge and consequently enhance the production capability of detectors for large area muon systems.
Deliverables:
-
D6.1: DLC production with C.I.D (CERN-INFN DLC machine)
-
D6.2: Industrial co-production of MPGD (micro-RWELL, Micromegas)
-
D6.3: MWPC- and GEM-based chamber production using industry-standard printed circuit boards with the option of integrated electronics.
T7: Longevity on large detector areas
At future experiments, the muon systems will be exposed to levels of radiation (rate of 10 MHz/cm2 and total collected charge of 10 C/cm2) never reached so, far for which they could suffer aging effects typically resulting in loss of efficiency, increase of the dark current and spurious signal rate. The severity of these effects increases with the integrated charge and dose, and it may depend on many factors, such as: the material of the electrodes, the gas mixture composition and flow rate, the gas layout distribution, etc. Accelerator longevity tests, including studies with eco-gas mixtures, at irradiation facilities are needed to identify possible aging effects by monitoring in time the main detector parameters and performance.
Deliverables:
-
D7.1: Studying the impact of integrated current and unknown gas-induced ageing effect on the long-term performance of the detector. Studies with eco-gas mixtures.
-
D7.2: Development and test of gaseous detectors operated with new recuperation and recirculation systems.
-
D7.3: Characterization of detectors constructed from industry-standard production methods in terms of ageing, environmental robustness (humidity, temperature cycling), mechanical stability (vibration, stress load) and gamma irradiation.
T8: New Detector Technologies
This task is dedicated to new ideas of detector structures, also coming from hybridization of established technologies, and from cross breeding between different detection techniques, and by its nature it is a task easy to link to other WPs. Just as examples, the use of pre-showers, or converters, within gaseous detectors; the modification of the field geometry to obtain better performance, such as in the Resistive Cylindrical Chamber, and its natural merging with a drift tube; the study of anisotropic resistive electrodes to boost counting rate performance on gaseous detectors.
Deliverables:
-
D8.1: Design, construction, and test of prototypes (area of 30x10 cm2) composed of Resistive Cylindrical Chamber (RCC), with different resistive materials and read-out electrodes.
-
D8.2: Hybridization of an RCC with a drift tube, and performance study
-
D8.3: Construction and test of a curved micro-RWELL prototypes with cylindrical and tubular shapes
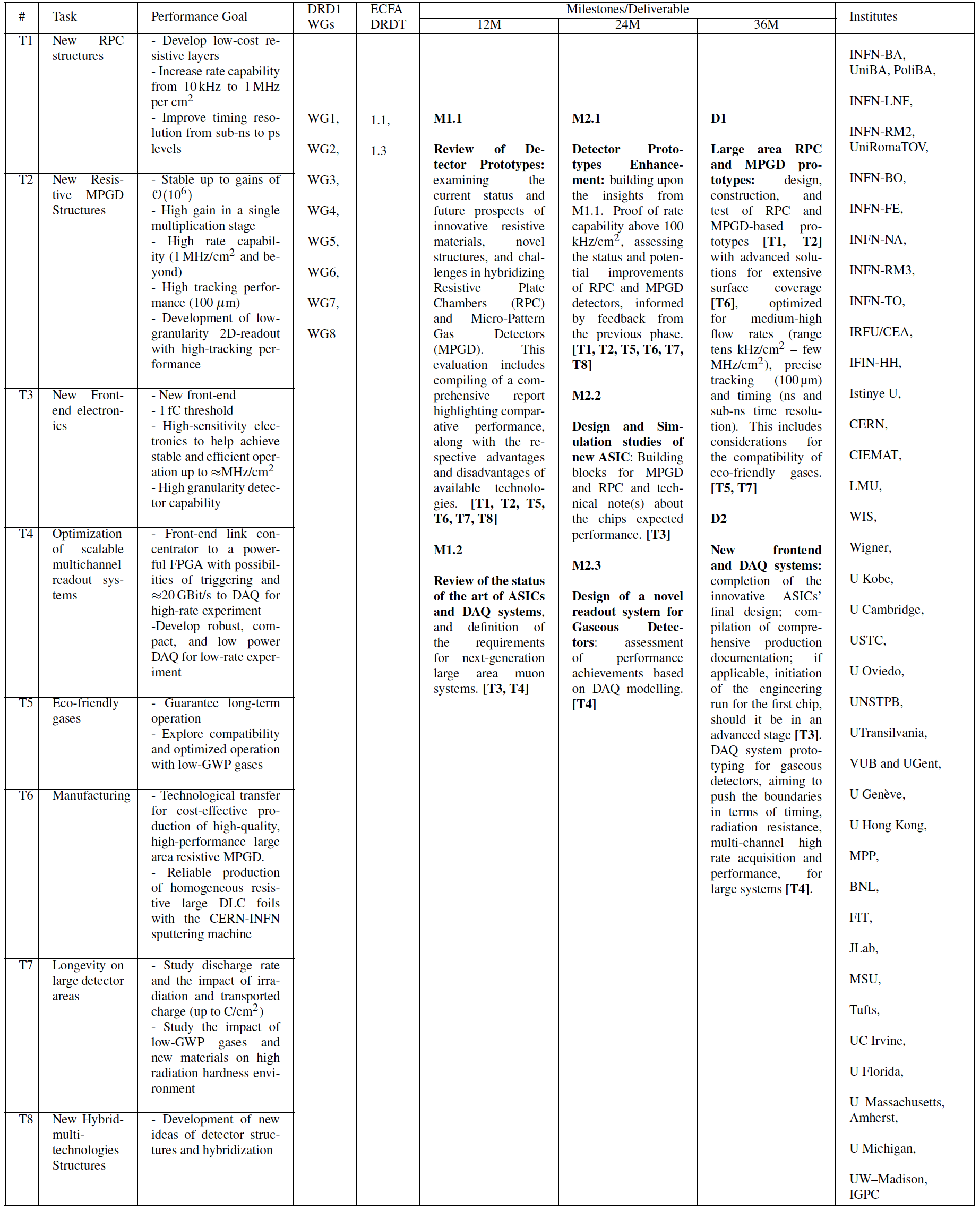