Gaseous timing detectors
WP Leaders and WP Project Leaders:
- Florian Brunbauer
- Ingo-Martin Deppner
- Diego Gonzalez Diaz
- Imad Laktineh
Contact e-mail: DRD1-WP7-leaders@cern.ch
WP7 proposal: link
DESCRIPTION OF THE WORK PACKAGE
The project aims to cover strategic R&D towards the development of stable, robust and longest-running gaseous based detectors capable of offering precise timing (from tens of ps up to a few hundreds), good space resolution (from hundreds mm to mm), different readout granularities (from mm2 to cm2 size pads) and layouts, high rate capabilities (from hundreds of KHz to MHz per cm2), large gain (single electron sensitivity). and with a modularity that will allow to equip large area (several m2) at practical costs. Sensitivity and response to charged particles and photon will be explored.
The long-term plans of this projects aims to match the requirements highlighted in the 2021 ECFA detector research and development roadmap. The relevant parts in terms of facilities requirements and recommendation are reported here. The proposed activities are covering the Detector Research and Development Themes DRDT 1.1 (Improve time and spatial resolution for gaseous detectors with long-term stability) and DRDT 1.3 (Develop environmentally friendly gaseous detectors for very large areas with high-rate capability).
This work package contains two projects:
-
WP7 Project A - High-rate, high-granularity precise timing with MPGDs
-
WP7 Project B - High-rate, large, precise timing (M)RPC
Each project contains a detailed R&D description, list of dedicated tasks, deliverables and timelines.
Main drivers from the facilities
Muon systems: A new generation of fast-timing GDs based on glass RPC, Multi-Gap RPC (MRPC), or fast timing MPGD (FTM) [Ch1-26], [Ch1-27] and PICOSEC [Ch1-28] are being developed, with a goal to achieve timing resolution of O(100/ps) and to reject off-time BIB hits. The main challenges at future facilities, particularly beyond 2030, include large area coverage with precision timing information (DRDT 1.1) to ensure correct track-event association, and the ability to cope with large particle fluxes using environmentally friendly gas mixtures (DRDT 1.3). Figure 1.2 summarises the main facilities, the proposed technologies to address the main challenges, and the most stringent conditions expected in muon systems.
Calorimetry: Ultra-fast picosecond-timing information with technologies like MRPC, PICOSEC and FTM can be used to resolve the development of hadron showers, resulting in a smaller “confusion term” and to improve jet energy resolution in hadronic calorimeters.
Photon Detection: Three generations of gaseous photon detectors have been developed: the GDs with converting vapours included in the gas mixture, open geometry MWPC with solid state CsI-photocathodes, and MPGD-based detectors with CsI-photocathodes. This historical development matches the need to provide progressively better solutions to the challenging requirements in this field, namely: (i) to reduce the photon feedback generated in the multiplication process which leads to spurious signals; (ii) to reduce the IBF rate because the ion bombardment destroys the proportional chamber and limits the lifetime of the detector (R&D line in common with TPC needs, DRDT 1.2) and (iii) to improve the detector performance in term of spatial and time resolution, along with fast response in order to open the way to high rate capabilities and precision measurements (DRDT 1.1)
Time of Flight Systems: Gaseous TOF systems are currently based on MRPC technology. The existing ALICE TOF detector, covering an area of ~150m2, has proved to be robust, stable and reliable and achieved a time resolution of ~60 ps Another fundamental MRPC parameter is the rate capability. For example, the large-area TOF in the CBM experiment at FAIR is designed to operate at particle fluxes up to 30 kHz/cm2 with a timeresolution of 80 ps . Other TOF systems, based on MRPCs, include eTOF wall for the CEE experiment at HIRFL-CSR and the TOF detector in the SoLID experiment at JLAB. R&D has to continue towards an ultimate time resolution of < 20 ps. This can be achieved by reducing the thickness of the gas gaps O(100 µm) and by increasing the number of gaps (up to order of tens) to maintain a high efficiency. In addition, time resolution below 15-20 ps is comparable to the avalanche jitter level, requiring novel very low noise front-end electronics. A rate capability up to 100 kHz/cm2, necessary for systems in high radiation environments, could be achieved by thinner (better signal induction), low resistive electrodes (order of 107 Ohm.m). MRPCs are currently studied. in order to fullil these requirements thanks to low resistivity, radiation hard ceramic electrodes.
In the case of MPGD, the front-end electronics needs to be improved with fast low-power amplification and continuous read-out. MPGD technologies for precision timing are FTM and PICOSEC with a goal to reach σ(MIP) ∼ 25 ps. Here the R&D is focused on scaling up to large area detectors which requires, in particular, to identify less expensive materials (radiators for PICOSEC) and very precise mechanical stability and uniformity (~10 μm flatness over 10 × 10 cm2). Radiation-hard photocathodes (CsI) are also needed for the anticipated particle fluxes. The main challenge for future R&D is to be able to keep uniform response, in terms of high rate capability and time resolution (DRDT 1.1), over a large TOF detector area while operating with eco-friendly gases (DRDT 1.3).
Trigger Systems: The muon tracking and triggering at a future hadron collider, such as FCC-hh, also requires large area coverage (~3000m2), while particle rates do not exceed 0:5 kHz/cm2 in the barrel and below 500 kHz/cm2 in most of the endcaps. The existing technologies for HL-LHC (MPGD, RPC, sMDT, sTGC) are adequate in most of these muon spectrometer areas. However, major R&D would still be needed for the very forward endcap region at a radius below 1 m.
Key technologies
For MPGDs, the main challenges remain large area, high rate, precise timing capabilities (PICOSEC, FTM), and stable discharge-free operation. They are addressed by developing resistive electrodes and exploring novel materials, amplifying structures, and detector architectures. Precise assembly at an industrial scale and integration of large area detector systems are additional items to be faced.
The focus for RPCs, MRPCs stays with the improvement of high-rate and precise timing capabilities, uniform detector response, and mechanical compactness. Exploration of novel materials and readout patterns, development of low noise electronics, optimisation of gas mixtures, including eco-friendly ones, and mechanical designs are the main future R&D.
Common challenges
ECO-gases: Therefore, reduction of the use of flourinated gases is fundamental for future GD applications. Flourinated gases will be banned in the new installations where alternatives are available, and emission from existing facilities needs to be minimised by checks, optimisations, and recovery systems. .. Possible alternatives to greenhouse gases(C2H2F4, SF6, CF4) should remain the principal focus of the future R&D
Ageing: Ageing phenomena constitute one of the most complex and serious potential problems which could limit or severely impair the use of gaseous detectors in unprecedented harsh radiation environments and lead to operational instabilities
Front End Electronics: Gaseous detectors require dedicated front-end electronics development, both discrete and ASICs, focused on specific applications and technologies, while addressing diverse requirements such as: fast timing, TPC readout, large input capacitance, low noise, cluster counting, input discharge protection, cross-talk reduction, large pixel size, compactness, low power consumption and detector integration.
TASKS
T1: Optimize the amplification technology towards large-area detectors
Optimization of the production processes of the selected amplification technology to assure response uniformity and reliable production processes for relatively large area coverage (several m2), identifying optimal modularity in terms of size and reduced dead areas.
T2: Enhance timing performance
Detector studies and optimization (e.g. primary charge, amplification, signal induction and electronics) to evaluate the intrinsic limit in terms of time resolution for the proposed technology.
For MRPCs, the impact of the number of gaps and the thickness of the gaps on the timing performance will be studied. Although several studies were performed in the recent past there is still a need to understand better this impact in particular with gas gaps of different thickness.
This work will be accompanied by a simulation study in order to foresee the best configurations for a given range of timing performance and then to test MRPCs built accordingly.
T3: Enhance rate capability
Detector studies and optimization (e.g. amplification and charge evacuation) to evaluate the maximum sustainable rates for the proposed technology.
This task will include two parts. The first one will be the construction of MRPC using different kinds of low resistivity electrodes. The second will tackle the timing performance when these MRPC are exposed to particle fluxes exceeding a few kHz/cm2.
T4: Spatial resolution and readout granularity
Enhance spatial resolution of precise timing detectors by optimizing readout granularity and develop readout solutions which can improve spatial resolution by resistive and capacitive charge sharing.
T5: Stability, robustness and longevity
Detector studies to reduce intrinsic limiting factors as space charge (rate capabilities), ion backflow (IBF) leading to ageing of photocathodes, and discharge probabilities at nominal working conditions.
T6: Material studies
Material studies and optimization (e.g. photocathodes and solid converters, resistive layers) towards the required longevity of the proposed technology.
T7: Gas studies for precise timing applications
Identifying mixtures with limited risks in terms of availabilities in the future (e.g. abundant, eco-friendly, and non-flammable).
The programmed abandon of the MRPC standard gas mixtures using high GWP gases like TFE and SF6 and their replacement by eco-friendly gases implies a thorough study of the impact of the new gases on the timing performance.
Different combinations of the new proposed gases will be studied and a comparison of the time resolution of each will be performed.
T8: Modelling and simulation of timing detectors
Development of modeling tools and techniques to optimize precise timing detector performance including the simulation of charge transport and signal induction and the influence of resistive detector elements.
T9: Readout electronics for precise timing
Single channel analog Front End optimization (e.g. robustness against discharges, high input capacitances, large dynamic range, fast rise time, sensitivity to small charges and low noise) for the proposed technology.
Scalable multichannel readout electronics for readout of detectors modules (hundreds of channels per module), exploring sampling and TDC readout solutions.
We propose to develop new electronics to read out large MRPC. The electronics will be built on the experience acquired from the ASICs developed recently by some of the groups involved in this WP. One of these ASICs called Liroc was co-developed by OMEGA group which is part of this work package. The ASIC is based on a very low-jitter preamplifier and very precise discriminator reaching less than 10 ps time resolution at charge of a few fC. In a common development on the T-SDHCAL project proposed within DRD6, we intend to upgrade Liroc by including a precise TDC similar to the one used in the HGROC ASIC for HGCAL CMS project with a time resolution better than 10 ps.
Multi-layer PCB that on one face will host the new ASICs and on the other the pickup pads or strips will be designed to read out the MRPCs. Several of these Large Active Sensor Units (ASU) will be put together to build large electronics boards to cover MRPC of 1 m2 or more.
A DAQ system communicating on the one hand with the ASICs of the ASU and on the other hand with computing units will be developed. It will be designed to be able to communicate with a few ASUs so a setup of a few MRPCs could be studied simultaneously.
T10: Precision mechanics and construction techniques
Identify needs, technological limits and potential improvement for each proposed technology towards granting precise mechanics (mm) over relatively large active areas (hundreds of cm2).
Develop technological solutions to minimize material budget of precise timing detectors including mechanics, amplification structure optimization and choice of charge conversion elements.
The standard method to build MRPC is based on using fishing lines with a diameter of 200-300 µm. This method implies the fishing lines to be well stretched and the plates are pressed on them so the gas gap width is constant and equals to the fishing line diameter. The fishing lines represents dead zones but also a source of noise. Using spot spacers to separate the plates is a good solution that is already used in some single gap RPC configurations where the gas gap is between 1 and 2 mm. This solution could be applied to the construction of MRPC but tiny insulating spot spacers of 200-300 µm are not commercially available in the industry and need to be produced on purpose. We intend to build MRPC with the two techniques and compare them to assess the advantages of using spot spacers in term of efficiency, noise and gas circulation performance with respect to the standard method.
T11: Common framework and test facilities for precise timing R&D
Implementation of common tools and techniques for the characterization of precise timing detectors across different technologies including MPGDs and (M)RPCs. Test benches for the detailed characterization of precise timing detectors with high precision timing references and spatial resolution for evaluating the uniformity of large-area detector modules.
Participating institutes:
- Aristotle University of Thessaloniki (AUTh)
- IRFU, CEA, University Paris-Saclay (IRFU/CEA)
- European Organisation for Nuclear Research (CERN)
- INFN, Pavia (INFN-PV)
- Jefferson Lab (JLab)
- Ruđer Bošković Institute (RBI)
- University of Science and Technology of China (USTC)
- Laboratory of Instrumentation and Experimental Particles Physics, Lisbon (LIP)
- Helsinki Institute of Physics (HIP)
- Institut de la physique des 2 infinis de Lyon (IP2I)
- Centro de Investigaciones Energéticas, Medioambientales y Tecnológicas (CIEMAT)
- Vrije Universiteit Brussel (VUB)
- Gangneung-Wonju National University (GWNU)
- Shanghai Jiao Tong University (SJTU)
- Organisation de Micro-Électronique Générale Avancée (OMEGA)
- Physikalisches Institut, Heidelberg University (HDU)
- Kyoto University (KU)
- Laboratório de Instrumentação e Física Experimental de Partículas (LIP)
- Tsinghua University (TSU)
- Shenzhen Institute of Advanced Technology (SIAT)
- Daegu Gyeongbuk Institute of Science and Technology (DGIST)
- Max-Planck Institute for Physics (MPP)
- INFN-BARI
- Roma Ter Vergata
- Hanyang University
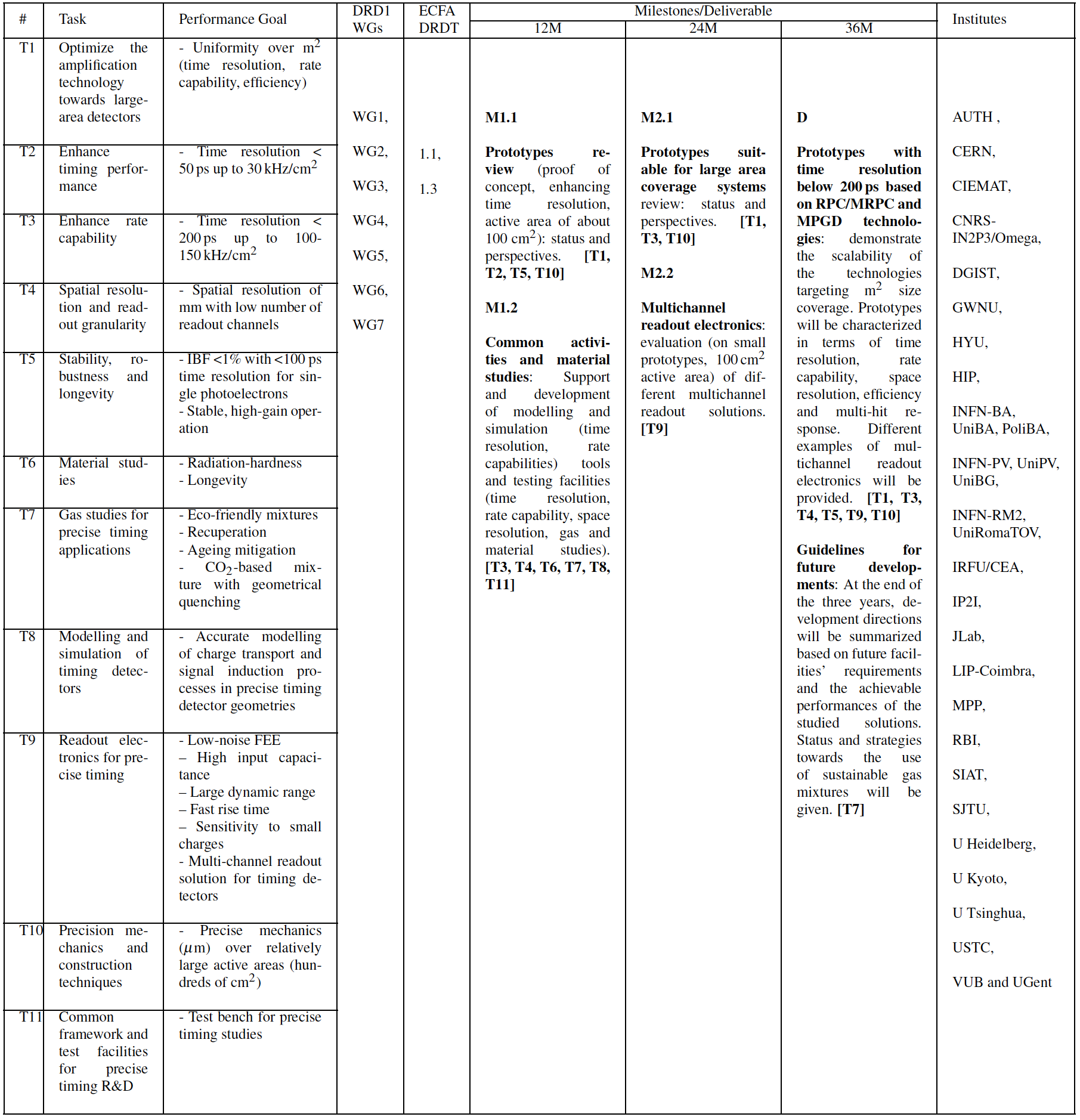